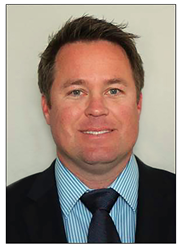
Victor R. Lange, PhD, JD, MSPH, ICP, CRC, CRA
AHMC Healthcare Director of Quality/Risk Management/Infection Prevention
In our experimental situation, use of the COVAIRE plastic barrier drape on the patient (emitter) can significantly reduce the amount of particles emitted and received.
MCDONOUGH, Ga. (PRWEB)
June 29, 2021
Encompass Group, LLC has funded and is presenting the following study using the COVAIRE plastic barrier drape (1) Severe acute respiratory syndrome coronavirus 2 (SARS-CoV-2) disease coronavirus (COVID-19) was first reported in Wuhan, China at the end of 2019 [1]. The virus has since spread across the globe, with over 83 million reported cases, and over 1.8 million deaths as of January 2021 [2]. Similar to previous viral disease outbreaks and epidemics, there has been a substantial burden of healthcare worker (HCW) infection. Probable reasons include a lack of adequate preparedness, a lack of institutional infection prevention and control measures, poor access to and compliance with personal protective equipment (PPE), and exposure to high-risk aerosol-generating procedures, such as endotracheal intubation, airway suctioning, and nebulization of medication [3].
Morbidity and mortality among HCWs infected with COVID-19 are suspected to be higher than that of the general population, possibly due to exposure to a higher viral burden during patient care [4]. Furthermore, the relatively higher estimated basic reproductive number (R0 ≈ 2-3) associated with SARS-CoV-2, exposure to asymptomatic carriers presenting with non-COVID-19 related illnesses, and unusual patient presentations, such as cerebrovascular accidents, Guillain-Barre syndrome, cardiovascular complications and skin rashes, further compound the risk facing frontline HCWs [5, 6].
A recognized key to transmission of COVID-19, and droplet infections generally, is the dispersion of bioaerosols from the patient (i.e., emitter). Increased risk of transmission has been associated with aerosol-generating procedures that include endotracheal intubation, bronchoscopy, open suctioning, administration of nebulized treatment, manual ventilation before intubation, turning the patient to the prone position, disconnecting the patient from the ventilator, noninvasive positive-pressure ventilation, tracheostomy, and cardiopulmonary resuscitation. The knowledge that COVID-19 subjects can be asymptomatic and still shed virus, producing infectious droplets during breathing, suggests that HCWs should assume every patient is potentially infectious during this pandemic.
Taking actions to reduce risk of transmission to HCWs is, therefore, a vital consideration for safe delivery of all medical aerosols. Guidelines for use of PPE (gowns, gloves, masks, face-shields, and/or powered air purifying respiratory) during high-risk procedures are essential and should be considered for use with lower-risk procedures such as administration of uncontaminated medical aerosols. Bioaerosols generated by infected patients are a major source of transmission for SARS CoV-2, and other infectious agents. In contrast, therapeutic aerosols do not add to the risk of disease transmission unless contaminated by patients or HCWs.
To mitigate this exposure risk during procedures, such as endotracheal intubation, various “aerosol boxes” have been designed, including by frontline HCWs [7, 8]. The majority of these boxes consist of a simple, three-sided, clear acrylic box with two armholes through which the patient airway can be accessed. The box is placed over a patient’s head and shoulders, clinicians place their hands through two large holes in the box to intubate the patient inside. In a simulation mannequin-based study where fluorescent dye and ultraviolet light were used to track dispersion of droplets and aerosol particles after a simulated cough, the “aerosol box” was shown to substantially reduce clinician exposure to aerosol particles [9]. However, in practice, these boxes have been found to hamper endotracheal intubation and other procedures due to the limited space and maneuverability they allow [10]. In addition, while such a device may contain the worst of the splatter, it can’t keep aerosols from leaking out. Because of concerns about the potential for airborne viruses to leak from the clear acrylic boxes, the Food and Drug Administration (FDA) revoked its Emergency Use Authorization (EUA) for these enclosures [11, 12, 13].
The airborne transmission route for SARS-CoV-2 is virulent for the spread of COVID-19 [14], as for SARS-CoV-1 [15]. At present, neither the aerosol viral load nor the minimum infectious dose of SARS-CoV-2 has been clearly established [16]. A viable virus can be emitted by an infected person by breathing or talking, without coughing or sneezing [17]: the louder the voice, the greater the quantity of aerosols emitted; furthermore, a small fraction of individuals behave as “super-emitters,” consistently releasing an order of magnitude more particles than their peers [18].
The aim of this study was to evaluate the performance of a specially designed, dedicated-use product: a customized plastic barrier drape—the COVAIRE plastic barrier drape—placed over a patient (emitter) during tracheal intubation / extubation procedures, as compared to no other “containment” barriers, and to document the relative impact on emissions to the receiver (HCW).
Methods
An experimental setup was developed, utilizing two mannequin heads with torso, positioned at 5-feet high, and at 10 inches from one another. An atomizer (TRIXIE Fogger XL) was utilized to generate distilled water fogging/seeding, set at a generating speed of 16.5 feet/sec from the emitter to the receiver head mannequin(s). This aerosol generating device was used to produce 2,500 times more particles (5 ml/min) than that of what a physiological cough would produce. A particle counter allowed us to evaluate the number of particles received on a mannequin head located at a distance of 10 inches, replicating the space between patient and HCW (e.g., anesthesiologist) faces during intubation.
The tests were carried out in an empty closed room of 40 feet2 without drafts, with mechanical ventilation turned off, and with entrance door closed and sealed at bottom with draft prevention tape. An optical particle counter (TROTEC PC2020) was used to reliably measure the size of particles in the air. The device utilizes an integrated measuring cell with laser (class 3R laser, 780nm, 1, 5-3mW). The particle counter sucks in air for an adjusted amount of time, thereby determining the amount of particles contained within the device after activation.
During the experiment, the aerosol generator was started at maximum power. The counter was started at the same time but with a 5-second programmed delay from the generator. The particle counter calculated the total cumulative particles aspirated on a volume of 1.2 liters. Counting was performed on 6 channels (0.3 µm – 0.5 µm – 1 µm – 2.5 µm – 5 µm – 10 µm) during 30 seconds of air aspiration.
After each measurement, the counter was reset to zero by the HEPA filter and the room, and ventilated by turning on the mechanical ventilation for 10 minutes to remove airborne particles. The Emitter-Receiver basic configuration was not changed during the entire experimentation: only face shields, masks, and COVAIRE plastic barrier drapes had been exchanged for the data acquisition.
A HEPA filter was used on the counter to reset the device to zero prior to each measurement. Particle sizes were stratified and counted in accordance to particle size, utilizing an optical counter set to channels 0.3 µm, 0.5 µm, 1 µm, 2.5 µm, 5 µm, and 10 µm. Reduction factors were adjusted in accordance to face shield with N-95 mask protection worn by the HCW/anesthesiologist (receiver) alone, COVAIRE plastic barrier drape on the simulated patient (emitter) alone, and then the double protection of emitter and receiver were calculated.
The aerosol was then generated by activating the emitter. The airflow speed at the emitting mouth was noted. The particle amount and the size distribution of the aerosol were measured at a distance of 10 inches (30 separate measurements).
When the receiver (i.e., HCW) wore only an N-95 mask, the particle reduction was 20.1% (p = 0.003). When the receiver wore a face shield with an N-95 mask, the amount of total particles was reduced 46.9%. With the protective COVAIRE plastic barrier drape covering the emitter (i.e., patient), and no receiver protective measures, the level of particles received was reduced by 96.8% (i.e., a reduction from emitter to receiver). The double-protection method (i.e., receiver face shield and N-95 mask plus emitter COVAIRE plastic barrier drape) allowed for even better results, a 98.2% particle reduction, but remained close to the protective COVAIRE plastic barrier drape over the emitter alone (96.8%). Even with small particle size emission (≤0.3µm), results were of the same order.
(1)The COVAIRE plastic barrier drape was chosen for evaluation as it is registered with the FDA in the regulatory category as a Class II exempt medical device, therefore this device does not fall under the revoked EUA.
Summary
In this simulation study with the COVAIRE plastic barrier drape covering the patient, and no provider protective measures, particle reduction was 96.8%. The double-protection method with face shield with N-95 mask plus the COVAIRE plastic barrier drape resulted in a 98.2% particle reduction on the provider, compared to 20.1% with N-95 alone.
Data Analysis
First, the amount of particles and the distribution of the particle size at a distance 10 inches were studied without use of the COVAIRE plastic barrier drape covering the patient.
For the experiments performed with the aerosol particle measurement instruments, the parameters studied and compared were: a) the impact of the presence of COVAIRE plastic barrier drape on the patient, b) the difference between protection of the receiver if drape use on emitter, face shield and mask worn on receiver, or both protection, c) the effects of different particle sizes on the results (0.3 µm – 0.5 µm – 1 µm – 2.5 µm – 5 µm or 10 µm respectively).
As part of our descriptive analysis, we utilized mean, median, and standard deviation for continuous variables. Distribution of each particle type was described by their means frequencies and percentages. A hierarchical linear regression was also utilized based on differenced in protection of emitter vs. receiver. For categorical variables, face shield, emitter, and aerosol particle < 0.3 µm were used as references. Sensitivity analyses were performed with comparison between the different experiments and subgroup analysis for micro (<5µm) and macro particles (≥ 5µm).
Results
All measurements were cumulative counts of different particles at the same distance (10 inches) during the same time (30 sec) after the same delay (5 sec), between emission and start of the particle counter on the receiver side. The air volume aspirated (1.2 l) was identical in all experiments.
The background level of particulate pollution on the aspirated air volume was standard in the experimental room, demonstrating a value of 16,229 particles (sd = 2,436). The mean concentration was 8,141 total particles/liter.
The aerosol produced has a distribution of particles, received at 10 inches, decreasing according to size. The average amount of particles measured at 10 inches without the COVAIRE plastic barrier drape on the patient, with 1,200,492 particles (sd = 192,120), with 72% particles received less than 0.3µm in size. The number of detected particles decreased with increasing particle sizes. This distribution was homogeneous in the different experiments: the linear regression model shows a constant decrease in the estimates compared to less than 0.3 µm particles (p<.001).
In our experimental situation, use of the COVAIRE plastic barrier drape on the patient (emitter) can significantly reduce the amount of particles emitted and received. The number of particles detected decreased significantly when the emitter was covered by the COVAIRE plastic barrier drape.
As expected, our results indicate more effective protection when the emitter is covered with a protective COVAIRE plastic barrier drape during intubation and extubation during aerosol-generating procedures (e.g., bronchoscopy, suctioning of the respiratory tract endotracheal intubation) on patients who are suspected of being infected with an agent for which respiratory protection is otherwise recommended (e.g., M. tuberculosis, SARS or hemorrhagic fever viruses).
Our experimentation is based on short and intense transmission. Transmission by aerosolization could also be achieved by long, low-intensity exposure, not tested here in our study.
Conclusion
Despite FDA EUA revocation for clear acrylic enclosures used as protective “intubation boxes,” our study demonstrated that when the specifically designed COVAIRE plastic barrier drape, that is registered with the FDA as a Class II exempt medical device, which is flexible, is tucked under the patient’s head and covers the patient body, HCW exposure to aerosolized particles is significantly reduced. Furthermore, due to the disposable nature of the COVAIRE plastic barrier drape, there is no need for disinfection of clear acrylic “intubation box” surfaces. The barrier is effectively rolled inward and disposed of according to standard infection-control and contaminant discard protocols. The COVAIRE plastic barrier drape does not create added infection risk. The evaluated COVAIRE plastic barrier drape can safely be used to reduce transmission of SARS-CoV-2 during intubation and extubation procedures.
References
1. Coronaviridae Study Group of the International Committee on Taxonomy of Viruses. The species severe acute respiratory syndrome-related coronavirus: classifying 2019-nCoV and naming it SARS-CoV-2. Nat Microbiol. 2020; 5:536–544. doi:10.1038/s41564-020-0695-z.
2. WHO. Coronavirus Disease (COVID-19) Situation Reports. Available at https://www.who.int/publications/m/item/weekly-epidemiological-update—5-january-2021.
3. Chan-Yeung M: Severe acute respiratory syndrome (SARS) and healthcare workers. Int J Occup Environ Health. 2004, 10:421-427. 10.1179/oeh.2004.10.4.421.
4. COVID-19 Scientific Advisory Group Rapid Response Report (2020). Accessed: Dec. 1, 2020: http://albertahealthservices.ca/assets/info/ppih/if-ppih-covid-19-hcw-risk-rapid-review.pdf.
5. COVID- 19: What proportion are asymptomatic? (2020). Accessed: Dec. 3, 2020: https://www.cebm.net/covid-19/covid-19-what-proportion-are-asymptomatic/.
6. Vetter P, Vu DL, L’Huillier AG, et al.: Clinical features of Covid-19. BMJ. 2020, 1470:m1470. 10.1136/bmj.m1470.
7. Aerosol box protects healthcare providers during endotracheal intubation (2020). Accessed: Dec. 8, 2020: http://sites.google.com/view/aerosolbox/home.
8. Chahal AM, Van Dewark K, Gooch R, Fukushima E, Hudson ZM. A Rapidly Deployable Negative Pressure Enclosure for Aerosol-Generating Medical Procedures. medRxiv 2020.04.14.20063958; doi: https://doi.org/10.1101/2020.04.14.20063958.
9. Canelli R, Connor CW, Gonzalez M, Nozari A, Ortega R: Barrier enclosure during endotracheal intubation. N Engl J Med. 2020, 382:1957-1958.
10. 1056/NEJMc2007589 10. Should we use an “aerosol box” for intubation? (2020). Accessed: Dec. 9, 2020: http://litfl.com/should-we-use-an-aerosol-box-for-intubation/.
11. FDA. Manufacturers of Protective Barrier Enclosures.
12. Simpson J.P., et al. Measurement of airborne particle exposure during simulated tracheal intubation using various proposed aerosol containment devices during the COVID‐19 pandemic. Anesthesia, 19 June 2020, 1-9.
13. Begley J.I., et al. The Aerosol box for intubation in COVID-19 patients: an in-situ simulation crossover study. Anesthesia, August 2020. 75 (8), 1014-1021.
14. Doremalen N, Bushmaker T, Morris DH, Holbrook MG, Gamble A, Williamson BN, et al. Aerosol and Surface Stability of SARS-CoV-2 as Compared with SARS-CoV-1. N Engl J Med. 2020; 382:1564–1567. doi:10.1056/NEJMc2004973.
15. Booth TF, Kournikakis B, Bastien N, Ho J, Kobasa D, Stadnyk L, et al. Detection of Airborne Severe Acute Respiratory Syndrome (SARS) Coronavirus and Environmental Contamination in SARS Outbreak Units. J Infect Dis. 2005; 191:1472–1477. doi:10.1086/429634.
16. Buonanno G, Stabile L, Morawska L. Estimation of airborne viral emission: Quanta emission rate of SARS-CoV-2 for infection risk assessment. Environ Int. 2020; 141:105794. doi:10.1016/j.envint.2020.105794.
17. Yan J, Grantham M, Pantelic J, Mesquita PJB de, Albert B, Liu F, et al. Infectious virus in exhaled breath of symptomatic seasonal influenza cases from a college community. Proc Natl Acad Sci. 2018; 115:1081–1086. doi:10.1073/pnas.1716561115.
18. Asadi S, Wexler AS, Cappa CD, Barreda S, Bouvier NM, Ristenpart WD. Aerosol emission and superemission during human speech increase with voice loudness. Sci Rep. 2019; 9:2348. doi:10.1038/s41598-019-38808-z.